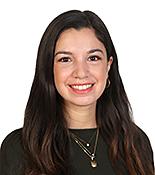
Nicoletta Petridou
Group Leader
ORCID: 0000-0002-8451-1195
EditCritical points and transitions in embryo development
Group Leader
ORCID: 0000-0002-8451-1195
EditMulticellular systems are dynamic systems that constantly change their morphology and associated physiology, an essential strategy for adapting to their environment and ensuring normal growth, development, and homeostasis. Microscopic mechanochemical interactions (molecular and cellular scale) underlie the macroscopic morphological–physiological changes (tissue scale). How this micro–macro link is established in vivo is the focus of our research. How can local microscopic patterns of cellular organisation trigger global macroscopic properties of tissues and what is the function of the latter in an organismal context?
Of special interest is dissecting the in vivo dynamics of this relationship, where gradual changes in local microscopic interactions can lead to abrupt transitions in global macroscopic mechanical properties, such as tissue stiffness or porosity. This nonlinear relationship relies on the presence of critical points, which from studies in physics have been shown to trigger symmetry breaking events, spontaneous order and to provide fitness to a system. A developing embryo is the most complex system known, exhibiting remarkable fitness. Just as critical points and transitions can explain the emergence of collective behaviour in everyday phenomena like magnetism and flocking birds, we aim to explore how they can explain the emergence and function of collective tissue properties during embryo development.
To address this, we utilise an interdisciplinary research line, combining tools from embryology, live imaging, genetics, optogenetics, computational imaging analysis, biophysics, and – in collaboration – theories from statistical mechanics. Our main system is the early zebrafish embryo at the onset of morphogenesis, when it transits from an amorphous pluripotent blastula to the multipotent gastrula undergoing extensive morphogenetic motion. By performing biophysical measurements, we have previously shown that the zebrafish blastoderm undergoes an abrupt decrease in tissue-scale viscosity, which is essential for its spreading. This abrupt tissue fluidisation is mediated by changes in cell–cell adhesion, where blastoderm cells gradually and subtly disconnect from their neighbours (see figure). Using quantitative approaches we showed that when cells reach a critical point in their connectivity, the tissue undergoes a rigid-to-floppy material phase transition, where rigid structures within the tissue abruptly disappear. A key observation is that the pluripotent embryonic tissues are positioned in the vicinity of this critical point of structural transitions, suggesting that the onset of morphogenesis operates at criticality.
By harnessing the physics of critical points, we are currently developing synthetic biology tools to control the vicinity of embryonic tissues to several critical points controlling a set of tissue physical properties (rigidity, confluency, porosity, topological order). This will allow us to address the role of collective behaviour in diverse developmental programmes, from cell fate decisions and cell proliferation, to metabolic reprogramming and cell mechanosensing.