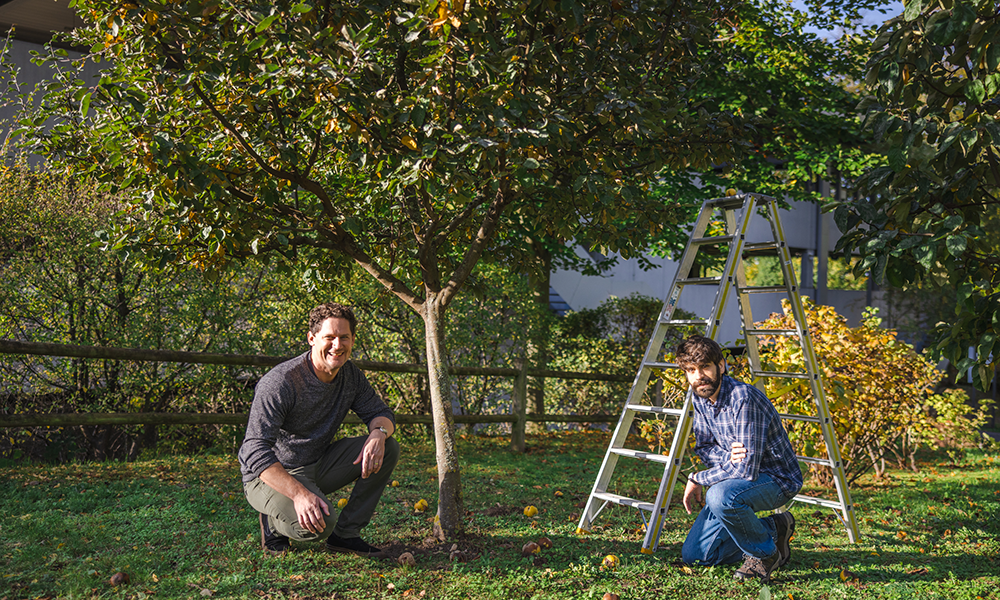
The power of a pesticide library
EMBL research groups apply molecular biology and its research tools to better understand agricultural pesticides
Issue 99
Kirill Kovalev, an EMBL Hamburg researcher, is studying the structure of an ancient bacterial molecule to help scientists control brain cell activity
Sunlight delivers precious energy that organisms capture using specialised molecular ‘solar panels’. Only two kinds of molecules can achieve this: chlorophyll-based proteins, which enable photosynthesis, and rhodopsins. Besides capturing solar energy, rhodopsins are also present in the retina and enable us to see.
In contrast to green chlorophyll-based proteins, which dominate on land, rhodopsins are mostly purple and found in aquatic microorganisms, especially ocean-living bacteria, archaea, algae, and even some giant viruses.
Kirill Kovalev, an EIPOD postdoc in the Schneider Group at EMBL Hamburg and the Bateman Group at EMBL-EBI, is fascinated by microbial rhodopsins. Trained as a physicist, he uses cutting-edge structural biology techniques to create molecular stop-motion visualisations showing how rhodopsins change their molecular structure to capture solar energy. With this knowledge, he designs new, more powerful rhodopsins that neuroscientists could apply as tools to control neuronal activity.
Why are rhodopsins and chlorophylls so colourful? The colour that we see is the light that these molecules reflect while absorbing all other wavelengths. For example, rhodopsins capture green and blue light and reflect purple wavelengths. Different types of rhodopsins reflect slightly different ranges of wavelengths, which gives them different hues of purple, violet, pink, and orange. Which wavelengths are absorbed or reflected is determined by the molecule’s structure. Rhodopsins have a simpler structure than chlorophylls and are believed to be evolutionarily older.
Rhodopsins’ ability to absorb specific light wavelengths not only gives them their pretty colours but also makes them scientifically interesting. In particular, one group of rhodopsins found in algae, called channelrhodopsins, is studied for its unique ability to trigger electrical activity in cells when exposed to blue light.
Scientists have adapted channelrhodopsins for human and animal cells, such that they can be used like an on- and off-switch for cellular activity. This technique, called ‘optogenetics’, was a game-changer for neuroscientists because it enables them to precisely and quickly stimulate selected neurons in the brain with just a flash of light.
Currently, scientists have only a few channelrhodopsins to pick from, which makes certain experiments difficult or even impossible. To address this, Kovalev is on the search for new, more powerful rhodopsins that could also be activated with other colours of light.
Using X-rays, he compares different rhodopsins found in bacteria, often in exotic places, such as salty lakes or glaciers. This lets him understand how tiny differences in the molecular structure determine protein properties and function, for instance, which colours a particular rhodopsin is sensitive to.
“Often, we are comparing almost identical rhodopsin variants,” said Kovalev. “A difference of just one atom is enough to change the molecule’s properties. That’s why we need to analyse them at atomic resolution.”
One advantage of these comparisons is that they let Kovalev predict which modifications would make rhodopsins responsive to new colours. Then, he and his collaborators can create these rhodopsins in the laboratory and test them.
What happens when a rhodopsin absorbs light? First, it gives rise to a complicated series of changes in the structure of the rhodopsin molecule. To visualise this process in detail, Kovalev uses a technique called time-resolved X-ray crystallography at EMBL Hamburg’s beamline P14. This experimental facility is adapted for particularly demanding crystallography experiments. X-ray crystallography enables Kovalev to take snapshots of a rhodopsin at specific timepoints after absorbing light and combine them into a stop-motion movie.
“Studying a molecule that undergoes so many changes within just milliseconds is extremely challenging. Besides, rhodopsins are membrane proteins. Such proteins are notoriously difficult to crystallise, which makes them even trickier to work with,” said Kovalev. “But I can do this at EMBL Hamburg’s beamline P14 at PETRA III, which is one of the best in the world for this type of experiments.”
Kovalev was one of the first at EMBL Hamburg to attempt a time-resolved crystallography experiment on a membrane protein. Before he could start experiments, the Schneider Group worked together, each member contributing different expertise, to make the beamline setup suitable for Kovalev’s project. Besides adjustments for membrane proteins, they installed a system to emit a flash of light to activate the rhodopsins, coordinated with the X-ray beam that would take a snapshot precisely at a selected timepoint milliseconds later.
In addition to the EMBL beamline P14, Kirill also performs experiments at the European XFEL, which allows for time resolution in the range of femtoseconds (one quadrillionth of a second).
“This combination of having a synchrotron and XFEL in one place is very helpful. Besides Hamburg, only Japan and Switzerland have a combo like this,” said Kovalev.
In the coming years, time-resolved experiments at EMBL Hamburg, such as this, will be further improved upon to observe how proteins work with even better time resolution. This will be possible with the planned upgrade of the synchrotron PETRA III to PETRA IV.
Kovalev, a physicist by training, first started to work on rhodopsins during his bachelor’s studies.
“What got me interested in biology was the opportunity to apply physics methods to solve unsolved problems. I like the thrill of being the first one to see how the molecule moves and understand how it works. I also like that my work can potentially help others in research and medicine,” he said.
One of the collaborative projects he’s involved in focuses on developing optogenetic tools to treat hearing loss. Kovalev, together with the Institute of Auditory Neuroscience at Göttingen University, aims to develop new rhodopsins that would help improve hearing quality in patients with cochlear implants.
In the new approach, rhodopsins will be placed into the cochlear auditory nerve using gene therapy. The nerve transmits information from the ear towards the brain, where it’s interpreted as sound. Neurons at different parts of the nerve respond to different sound properties, such as pitch or intensity. A new generation of cochlear implants produces patterns of light stimuli that activate these different parts. By encoding sound information with light, these newer implants could stimulate the cochlear nerve with increased precision, allowing patients to better hear fine differences between sounds.
This wouldn’t be possible without new powerful and fast-acting rhodopsins that could manage the complexity of quickly changing sounds around patients.
This project is just one example of how clinics could use designer rhodopsins. New rhodopsins might offer new applications in other areas as well.
“I believe the unique structural data we decipher will serve not only to help us understand fundamental principles of light energy use and reveal new cellular processes on Earth,” Kovalev said. “It will also contribute to developing rhodopsin-based biotechnology and medicine.”
EMBL research groups apply molecular biology and its research tools to better understand agricultural pesticides
The CABANA project was born out of a desire to strengthen bioinformatics capacity and accelerate data-driven biology in Latin America.
Click here to download a quick overview of all the articles included in this digital issue of EMBLetc.