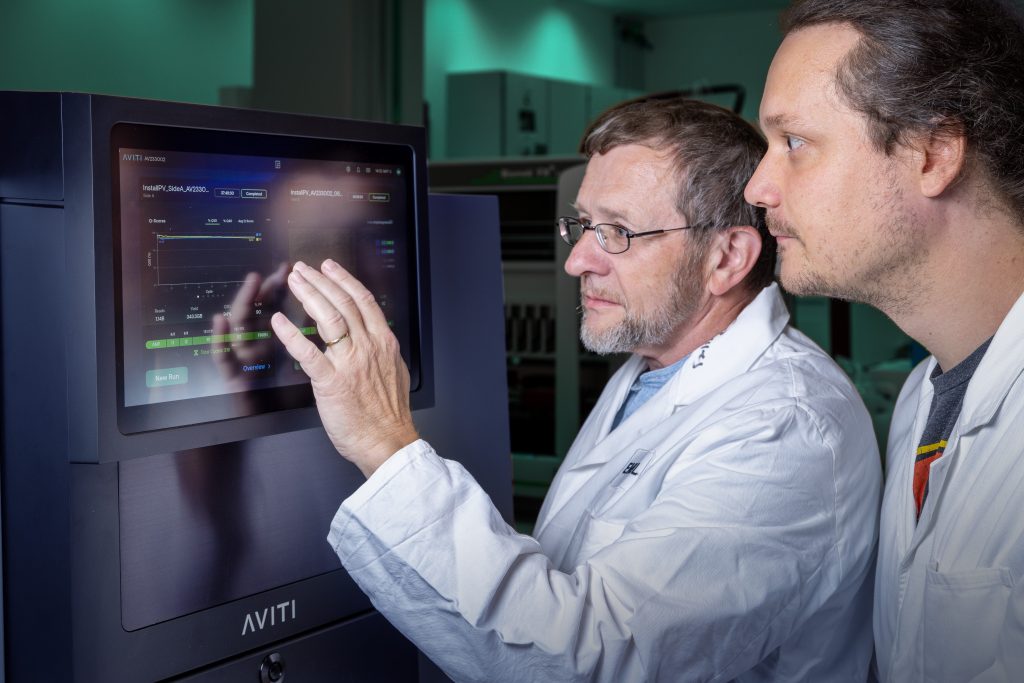
Embracing the genome sequencing revolution
EMBL’s Genomics Core Facility provides end-to-end support to researchers across Europe and beyond and stands at the forefront of scientific breakthroughs.
Issue 101
EMBL developmental biologists – with help from other disciplines – pursue the significance of time, timing, and transitions in organisms during their development
A bassoon’s rich timbre breaks the silence of a darkened auditorium, followed closely by flutes, violins, French horns, and a variety of percussion instruments. Singers enter the stage in costume, following precisely choreographed movements, their voices blending in perfect symphony. A spectacular operatic piece is underway.
At the heart of this performance is a network of dynamical systems – systems that evolve over time according to precise rules. Unseen cues from the conductor and interactions between instruments and performers combine to create a magical performance that could have easily devolved into chaotic noise. And the key elements that govern this system are: time, timing, and transitions.
The same three principles hold true for living systems, where even at a molecular level, thousands of processes happen at once – millions of tiny unseen operas. Developmental biologists in particular have long appreciated the critical importance of when things happen, at what pace, and how they correlate with the major transitional events of development.
In living systems, there is an arrow of time – a history. For decades, scientists have recognised the importance of this notion of time, timing, and transitions in living systems. The difference these days is that technology can now not only make this ‘time and timing’ visible by revealing oscillations and rhythms, but scientists can also manipulate and therefore study these concepts in developing organisms.
For example, a snapshot of a living system is unable to reveal ‘timing’. Instead, one must observe the systems over a long time. Over the past 10 to 20 years, this is why EMBL and other research institutes have been investing in finding ways to make timing visible, with microscopy and other tools that help us see the rhythms and associated dynamics within cells and within organisms.
The progress in theory, imaging technology, and techniques like microfluidics, have given scientists ways to study developing systems in a much more dynamic manner – yielding findings that could even be drivers for preventing and treating developmental diseases and disorders. However, this research is fundamental, with the central goal of gaining a clearer understanding of living systems and the varied internal and external cues that provoke changes in form, function, and behaviour.
Dynamical systems are not new to Soham Basu, a PhD student who moved to EMBL from Kolkata, India, transitioning from studying astronomy and theoretical physics to doing hands-on experimental biology in Aissam Ikmi’s research group.
Physicists have long applied dynamical systems to studying particles, or ensembles of particles, whose states vary over time, and this was the part of theoretical physics that most captivated Basu.
From his undergraduate days of coding, running simulations, and debugging theoretical suppositions, Basu moved on to observing the early morphogenesis of the starlet sea anemone Nematostella vectensis in the lab. Nematostella, an evolutionarily ancient, simple, yet distinctive genus, doesn’t exhibit signs of ageing and has regenerative capabilities.
Nematostella constantly expand and contract while water is pumped through their body cavity. Basu wanted to understand how the anemones’ very flexible skeletons couple with surrounding tissue and how the combination dictates the organism’s tube-like shape. On small time scales, such as seconds, the continuous pumping of water doesn’t have much impact on Nematostella’s size, but it underlies their flexibility. However, on a longer time scale, the anemones keep growing incrementally, while also becoming less flexible. Basu refers to this phenomenon as a ‘bridging of the scales’.
With support from EMBL’s Advanced Light Microscopy Facility team and its microscopes, Basu follows the growth trajectory of Nematostella larvae over a 36-hour period, finding ways to zero in on specific points in the growth process and at key locations of their developing bodies and explain the incremental biophysical process that stabilises the shape of the sea anemone at each step.
“At this point, we have a beautiful story of how interactions are happening,” Basu said. “And, in a way, I’m coming full circle, now collaborating with a theoretical biophysicist, who is well known for his work in morphogenesis.”
Each of us begins life as a single cell, which then divides into a mass of undifferentiated cells. Bit by bit, these undifferentiated cells get assigned functions and positions, and take these up to give rise to a specific shape per a body plan. This basic understanding is at the crux of the work of several researchers in Alexander Aulehla’s research group, including Simona Gioè and Sarkis Tafnakaji, both PhD students; and Simon Knoblich, a trainee who came to EMBL from medical school.
During development, each cell needs to know what to do and when to do it in coordination with everything that is going on. Gioè, Tafnakaji, and Knoblich are studying these timing cues and the larger impact they have on an organism’s development.
“In my research, I am looking at the timing of somite formation (the precursors to vertebrae) and trying to figure out what else is being controlled by this timing – is it just the time when things form, or does it influence the shape they will have?” said Gioè, who comes to EMBL from Italy.
Gioè uses a technique called microfluidic entrainment to manipulate the tempo of a ‘segmentation clock’ that dictates the rate of skeletal formation in mice embryos. Microfluidics involves the precise control and manipulation of flows with miniaturised devices. Using such a system, she periodically flushes the embryo with drugs that slow down or speed up development and then observes developmental consequences, such as changes in shape in the developing mice. In her current work, she looks specifically at signalling via the Notch pathway that not only is central to normal development but has been connected to tumour development.
Gioè follows skeletal development over 24 hours, taking images every 10 minutes. She then uses these images to reconstruct the time series and better understand the interconnectedness of the dynamics occurring at any given point. With this method, she is able to observe something not visible normally because it happens inside the uterus. The microfluidics bring it ‘ex vivo’ so she can capture a ‘sweet spot’ of imagery to see otherwise unknown nuances and analyse the dynamics in a manageable way.
Likewise, Knoblich, who comes to EMBL from Austria, is applying this same microfluidics approach to Japanese rice fish (medaka) for his research. He hopes to better understand the segmentation clock in a non-mammal vertebrate model.
Medaka embryos usually develop in the brackish water of rice fields in Japan. While the average water temperature is 28°C, embryos can experience seasonal and daily temperature fluctuations ranging from 10°C to 35°C. Despite these drastic conditions, medaka embryonic development has been shown to be remarkably robust. Knoblich is trying to gain an understanding of the segmentation clock, which is typically set to produce a new segment or somite every 80 minutes at 27°C. This is a faster model organism than the mouse, and comes with a wide array of genetic tools that offer Knoblich a variety of options for manipulating and viewing the transparent fish embryos.
In another project, Knoblich looks specifically at the very first stages of embryo development when medaka and other teleost (related ray-finned fish) embryos spread over their yolk in a fairly short span of time, undergoing significant morphogenetic and developmental changes. Knoblich is particularly interested in the collective calcium signalling that occurs in waves and that traverses throughout entire embryos at this stage.
“What I’ve found interesting is the comparative nature of this research,” Knoblich said. “It’s why I’m looking at doing similar experiments with killifish that experience diapause, a condition which suspends development and allows them to survive annual dry periods and environments hostile to other fish species. Despite being closely related to medaka, killifish go through very different early developmental stages that can span days to months (as opposed to hours in medaka), for reasons, and with biological mechanisms, not entirely understood yet.”
Tafnakaji’s work also is connected to Knoblich and Gioè’s research projects in that he wants to know how the dynamic signalling involved in somite formation instructs the developmental progress and subsequent differentiation.
Tafnakaji, who grew up in Armenia and Syria and came to EMBL after R&D research at AstraZeneca in Sweden, was also inspired by the segmentation clock. Wave-like signals across the developing tissue provide many cues for the cells. His work explores which of these cues are most relevant for the cells to synchronously and precisely form somite structures. Working with Gioè, Tafnakaji explores also how the change of timing in cellular communication can instruct the patterning of a developing embryo body.
Understanding these aspects of cell communication helps inform basic questions like, ‘If you have a group of identical cells, what are the different ways we might instruct them to create new, different types of tissues or structures?’
“This is curiosity-driven fundamental information that will help us later in realising our full potential in any sort of field in which cells are the building blocks, such as tissue engineering or cellular therapeutics,” Tafnakaji said. “This can be foundational work for anything we want to do that is cell-based.”
Just as Knoblich looks specifically at the very first stages of embryo development and the collective waves there, so do the researchers in Nicoletta Petridou’s group at EMBL Heidelberg.
The survival of the embryo is critically dependent on certain key transitions that occur early in development. Lena Schindler and Camilla Autorino, PhD students in the Petridou group, are focusing on a very short transitional window in zebrafish embryo development that may provide important clues to the biophysical interactions that shape this process.
Schindler and Autorino are exploring the biological functions of tissue transitions in development – work inspired by Petridou’s postdoc research at the Institute of Science and Technology, Austria. Petridou joined EMBL in 2020 and is originally from Cyprus. While observing the very first movement that embryonic tissue undergoes during development, she noticed a rapid transition where the tissue goes essentially from a solid to fluid state, and the cells’ collective resistance to flow abruptly drops.
The scientists describe this as a fluid state because the material state is measured based on its viscosity. Initially, when pipetted, the tissue shows high resistance and doesn’t quite “flow” up the pipette (like honey). Once the transition has happened, it is much more deformable and moves up the pipette quickly (more like water). Later, it changes back to a less deformable state.
Petridou’s work brought her in contact with Bernat Corominas-Murtra, a physicist at the University of Graz, Austria, who was also studying phase transitions, albeit not in biological systems. Petridou applied a mathematical framework from 1864 that used the concept of networks to understand real material properties of embryonic tissues. This subsequently helped shape her research direction.
“Imagine an iron bridge composed of lots of bars. You don’t have to remove all the bars for the bridge to collapse; it may only require a few bars being removed,” Petridou explained. “So, then, instead imagine this breach is happening in a network of cells and links between cells. As links are removed, the whole network becomes unstable, vulnerable to breaking.”
Petridou soon found that the minimum number of connections required to break a network, as predicted in the mathematical theory from 1864, matched what she found to be true in tissues as well. And rather than activity just within the cell, a cell’s connections to other cells determined the key solid-to-fluid transition of the tissue.
This approach has informed a lot of the Petridou group’s work on critical transitional moments in embryo development, where tiny cellular manipulations can have a tissue-scale effect, simply by disrupting the synchronicity between biological processes. As connections between cells change, transitions occur because collective tissue properties are changing too. The group is still investigating if and how cells respond to their environment and its changes.
“I think this is what is so interesting; you don’t have a steady state,” said Autorino, who joined Petridou’s group in 2020 and is originally from Italy. “The system keeps changing. In 10, 15, or 20 minutes, you create a new normal. And then it changes again.”
In that 10-minute period when zebrafish embryos are just beginning to set up their body plan, the tissue of the future embryo spreads and starts to cover the yolk while the yolk ‘domes’ upward into it to assist the process, as seen in the video here. Schindler, who came to EMBL from Austria, wants to better understand how cells work together to form tissues, and uses this key transition to understand how the synchronisation between cell divisions in the embryo (or lack thereof) can help regulate this process.
“Basically, I draw connections from the spaces between the cells,” Schindler said. “I can follow along as they divide cell by cell, sorting cells that are connected and creating maps that our mathematical collaborators then can use to compute correlations.”
Autorino’s work, on the other hand, focuses on a very specific region of the embryo that is the first to have its ‘fate’ determined; it marks the first signalling events happening in the tissue. As this happens, Autorino also observes mechanical and material property changes. Consequently, she aims to characterise how these mechanical and material properties affect what the cells ultimately transform into.
As recently as five years ago, one would be hard-pressed to find scientific literature about applying phase transition research in developing systems. But this approach has even broader applications. Metastasis in cancer cells, Petridou explains, is basically a transition of a solid tumour to a gas because the density of the cells becomes so sparse. On a much larger scale, avalanches, ecosystem transitions, and climate changes are also ripe for studying in this way.
The overlap between many of these research projects seems inevitable. Transitions depend on the synchronisation of multiple systems, and all such processes are controlled in space and also in time.
“There’s a very fundamental way that life is organised, and the notion of time and timing is at the heart of it,” said Alexander Aulehla, the head of EMBL’s Developmental Biology unit. “Within our bodies, we have different rhythms in different parts of our bodies, and these are coordinated.”
Aulehla is in turn fascinated with this natural synchronicity, seen across scales in biological systems.
“Even after an opera, think of the way a standing ovation takes over an audience,” he said. “You are just part of this moment that becomes synchronised with no maestro directing you. And that’s the kind of coordination that is happening between cells in every early embryo.”
EMBL’s Genomics Core Facility provides end-to-end support to researchers across Europe and beyond and stands at the forefront of scientific breakthroughs.
Promiscuity is critical for nourishment. How? This question lies at the focus of research by the Löw Group at EMBL Hamburg. Using structural biology methods, they explore how specialised molecules located in the cell membrane allow cells absorb nutrients from their environment.
Click here to download a quick overview of all the articles included in this digital issue of EMBLetc.