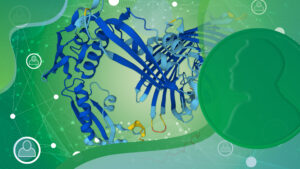
Read the latest Issue
As EMBL Hamburg celebrates its 40th anniversary, we explore the past, present and future of the incredible world of crystallography.
Just over a century ago, physicist Max von Laue pioneered a discovery that shone new light on the structure of our world. By firing X-rays at crystals, he revealed a pattern of remarkable spots that proved that X-rays are high-frequency waves. But by doing so, he also unearthed another puzzle: why did the X-rays scatter in such a regular fashion? The race was on to make sense of the story that von Laue’s two-dimensional pattern told. The father-and-son team of William and Lawrence Bragg focused their attention on the nature of the crystals themselves, and they realised that by studying the way the X-rays reflected, the pattern could be transformed into a three-dimensional model of the molecules constituting the crystal. They found that these molecules formed a lattice with atoms arranged in layers throughout, describing them as “like soldiers in a parade or the pattern of a wallpaper”. By working backwards from the pattern they were able to mathematically reconstruct the structure of the molecule, each ray relaying a tiny package of information about the molecules within. Crystallography was born.
Fast forward 100 years, and it is difficult to imagine where natural science would be without the field of structural analysis. Since the initial experiments that revealed simple structures of molecules such as salt and diamond, the technique has been used to analyse those of increasing complexity, not just matter that is naturally crystalline, but anything that can be induced to be crystalline – including proteins and viruses. Understand the structure of a molecule, and you can begin to understand its function, and the technique provides a means to learning why and how genes are turned on and off, how cells defend themselves against invaders, and the nature of the switches that initiate cell division. Indeed, crystallography has played a key role in countless stunning discoveries, including the structures of DNA, penicillin, myoglobin, the ribosome and, more recently, whole viruses.
Just as when Galileo first trained his primitive telescope on the night sky and so changed the way we view and understand the cosmos, X-ray diffraction allows scientists to see what was previously invisible, at the molecular level. But as with the development of early telescopes there were many hurdles still to come, and as molecular biology advanced, the limitations of early light sources such as laboratory X-ray tubes were exposed. But a crucial breakthrough came in 1970, when Ken Holmes and Gerd Rosenbaum of the Max Planck Institute for Medical Research in Heidelberg published a study in Nature that investigated the mechanisms of insect flight muscles. The work, which was carried out at the German Electron Synchrotron (DESY) in Hamburg, included a smudgy photograph of a diffraction pattern that sparked worldwide interest: proof that synchrotron radiation can be used to study biology.
What was previously the toolbox of physicists became useful for molecular biologists too, and many more biological secrets were set to come within the firing range of structural analysis. Holmes mobilised some of the biggest names in science, and his vision led to the establishment of facilities to run structural biology experiments at the DESY campus – the birth of EMBL’s first outstation, in Hamburg, in 1974 – and the beginning of a long and celebrated partnership with the research centre that continues to this day.
In a synchrotron, particles are first accelerated along a linear track before being passed into circular machines, where magnets force them to move around a circular path. As they do so, light in the form of X-rays is radiated outwards, much like how you might lose the contents of your pockets while sitting on a roundabout at the fair. This synchrotron radiation can then be channelled through a series of optics and mirrors known as a beamline, firing an extremely intense, narrow beam of X-rays at the crystal. This beam – many magnitudes brighter than sources used to take X-rays at the doctor’s surgery – reveals much greater structural detail from weakly scattering samples such as protein particles. Today, biologists are amongst the greatest users of synchrotrons and the instruments used are larger, more complex and more precise than the pioneering scientists of yesteryear could ever have imagined.
Since 2009, DESY’s campus has been home to PETRA III – one of the most brilliant storage ring-based X-ray radiation sources in the world. Here, structural biologists from around the world have access not only to top-level instrumentation, but also to sample preparation and characterisation facilities, small angle scattering beamlines, data analysis software, robotics, as well as scientific and logistical support. The structures of larger and more complex proteins can be tackled with greater precision, fewer resources and less time – many remotely from the comfort of the user’s office.
Today, biologists are amongst the greatest users of synchrotrons
But for all these technological developments, there is still one crucial limiting factor when it comes to crystallography: crystals. Unfortunately, there is no universal recipe for producing them. Many crystallographers spend weeks, months or even years trying to crystallise a protein, gently tinkering with different concentrations of buffers and salts to seek out the optimal conditions. As our understanding of biology advances so too does our ambition to decipher the workings of larger complexes, such as membrane-bound proteins that mediate molecular traffic coming in and out of the cell. And it is exactly these molecules that are exceptionally difficult – if not impossible – to crystallise due to complexities in the scattering process that cannot be corrected using data analysis. Or, equally frustrating, some produce multiple microcrystals – too small to use in X-ray analysis or too weak to survive damage from radiation.
Since 2009, DESY’s campus has been home to PETRA III – one of the most brilliant storage ring-based X-ray radiation sources in the world.
New techniques such as serial femtosecond crystallography are offering the tantalising prospect of overcoming some of these hurdles. Massive machines called free electron lasers (FEL) accelerate electrons along a linear track until they produce very short and intense X-ray pulses. From this, pulses of light lasting just 50 femtoseconds (20,000 times shorter than a nanosecond), and with a peak energy one billion times greater than the most powerful synchrotrons, smash crystal samples down to a micron in size to smithereens almost instantly. But crucially, if the X-ray pulse is short enough, it can pass through the crystal before it has time to explode – producing a clear diffraction pattern, with no radiation damage.
Researchers using the FLASH FEL on the DESY campus – a smaller version of the future European XFEL due to power up in 2017 – demonstrated this ‘diffraction before destruction’ principle, meaning that images can be collected faster than the X-rays destroy the sample. Studies using the Stanford-based SLAC National Accelerator Laboratory FEL have taken this a step further, with researchers recently solving previously unknown structures such as cathepsin B – an enzyme found in the sleeping sickness parasite Trypanosoma brucei. But availability of beam time is very limited, and a huge challenge lies in producing the massive amounts of sample needed for experiments to succeed.
These were challenges EMBL Hamburg group leader Thomas Schneider had in mind when leading a study to solve the structure of cathepsin B for a second time. But a simple replication exercise this was not. Instead of using a FEL, he wanted to explore the complementary potential of the latest generation of synchrotrons. Back when EMBL began to plan the construction of the next generation of beamlines at PETRA III, Schneider’s vision was to develop instruments capable of measuring micron-sized crystals, mobilising the power of third-generation synchrotrons to determine the structures of the tiniest crystals composed of the largest repeating units. Some six years since the construction of the beamlines began, they managed to do exactly that. In a paper published in 2014 in the International Union of Crystallography (IUCr) Journal, the team of scientists from EMBL, the Centre for Free-Electron Laser Science (CFEL) and the universities of Hamburg and Lübeck, resolved the crystal structure of cathespin B by analysing microcrystals of the enzyme, this time using synchrotron radiation.
A typical serial femtosecond crystallography experiment at a FEL involves flowing a suspension of microcrystals across a fine and intense X-ray laser beam – much like the flow of ink from an inkjet printer – which results in hundreds of thousands of single diffraction images. This principle was adopted for use on the EMBL microfocus beamline P14 at PETRA III. But instead of shooting the crystals across an X-ray beam, a small volume of cryo-cooled crystalline solution containing around 5000 crystals was mounted in a standard nylon loop, which was then scanned by the PETRA III beam.
The results are hugely encouraging: although the resolution doesn’t (yet) quite match that of the data collected at the FEL, the team is convinced there are many other benefits, including less resources needed, and greater availability and accessibility of equipment. “Our experiment used a lot less sample than that needed for the FEL and measuring the crystals at the synchrotron could be a useful first step to check and optimise the quality of your sample, before having to produce large amounts for FEL experiments,” Schneider explains. “The set-up is conceptually simple and can be applied to other state-of-the art synchrotrons – in comparison to the restricted access to FEL facilities,” adds Gleb Bourenkov, EMBL Hamburg staff scientist and project leader at beamline P14.
Crucial for the success of the project was the high precision diffractometer developed together with colleagues at EMBL Grenoble and the micrometer-sized high intensity X-ray beam produced at PETRA III. “We have just made optimal use of what we had available, but none of this would have been possible without our close and productive working relationship with colleagues at EMBL Grenoble and DESY,” says Schneider, who points out that background “noise” can be countered by reducing the beam size to exactly that of the crystal, delivering a clearer signal and higher resolution data. The technique, known as serial synchrotron crystallography, will be available as a standard method on P14 for visiting users later in 2015.
This work has added to the palpable sense of excitement ahead of the opening of the European XFEL in two years time. On the other side of the DESY campus, stretching some 3km through underground tunnels into the neighbouring federal state of Schleswig Holstein, the most powerful FEL in the world is currently under construction. “The ultra-fast flashes produced by the European XFEL could make it possible to take pictures of single protein molecules without the need for crystallisation, or watch molecular reactions in real time,” says Matthias Wilmanns, Head of EMBL Hamburg. “For example, international teams have already managed to stimulate and record structural changes in light sensitive proteins involved in photosynthesis. The possibilities are seemingly endless and truly mind boggling.”
Collaborative projects in anticipation of this new research arsenal have already begun to exploit the remarkable potential for measuring three-dimensional structures and dynamics of single bioparticles. One example is an initiative to standardise protocols for sample preparation, provide novel sample delivery methods, and develop the first comprehensive software package to interpret the data is being coordinated by EMBL Hamburg group leader Victor Lamzin. Called “Laseromics”, it brings together experts from EMBL Hamburg, University of Osnabrück and Moscow institutes – including Moscow State University and the Kurchatov Center.
Another sees Wilmanns and Schneider team up with Henry Chapman, a physicist at CFEL, and two groups in Moscow, for a project aimed at developing combined applications for synchrotron radiation and FELs in molecular medicine. “We are seeing some great collaborations and innovative projects being established across the campus between groups working at the synchrotron and XFEL and we are sure this spirit will continue,” adds Wilmanns. “During the past few years we have seen how important it is to have a sample preparation laboratory directly next to the PETRA beamlines to ensure effective and timely use of precious protein samples. We are eager to apply our knowledge gained from working at the synchrotron to new challenges ahead at the European XFEL.”
Looking for past print editions of EMBLetc.? Browse our archive, going back 20 years.
EMBLetc. archive