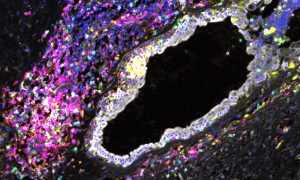
Read the latest Issue
Until recently, the composition of microbial communities was believed to be largely shaped through competition. Now, scientists at EMBL Heidelberg have discovered that, while substantial competition for resources exists within larger communities, smaller groups regularly form within them whose members behave more socially.
In recruitment, candidates have better chances of being hired if they bring skills to the workplace that complement those of other team members. Similarly, collaboration between different member species is crucial to the way microbial communities form.
The idea of cooperation as a decisive factor in community formation isn’t completely new. Cooperative actions, like the exchange of metabolites, have previously been suspected of having an effect on microbe community formation. However, metabolic exchanges are difficult to discover.
“Individual species metabolic modelling has been going on for a long time,” says Kiran Raosaheb Patil, lead author of the present study, published in PNAS. “However, once you put more than one bacterium together, it becomes very difficult. Bacteria can start interacting, they secrete and use different metabolites which, unlike DNA or RNA, cannot be easily traced back to a single source.”
To meet this challenge, Patil’s team developed a computer model that allows predictions about what happens when different species come together. “It is a hypothesis-generating machine,” says Patil, “that can enumerate all possibilities of interaction. It traces the different things that different bacteria do with the same metabolite, and which metabolites are most likely to be exchanged.”
It traces the different things that different bacteria do with the same metabolite, and which metabolites are most likely to be exchanged.
The team’s modelling approach, applied to 800 previously described microbial communities, is called ‘species metabolic interaction analysis’, or SMETANA, for short. To develop it, the scientists used a compilation of previously published 16S ribosomal RNA sequences to identify 261 species living within 1297 communities. To find out whether some of these species depend on each other, the team analysed which of them gather in so-called co-occurring sub-communities – groups of species that regularly assemble within a larger community. Using metabolic models for each of the 261 species, the researchers reconstructed the metabolic processes at play within these communities to make predictions about both metabolic competition and cooperation between members.
“Competition,” says Patil, “is unavoidable. In a natural environment, resources will always be limited and some species will always end up competing for the same resource.” To quantify that competition, the scientists came up with a metric they call metabolic resource overlap (MRO). MRO compares the nutritional components that each of the present species absolutely needs to survive with the components that all of them need to survive – the ones they might compete for. Noticing that communities as a whole are closely genetically related and also show high MRO, the researchers concluded that they must have similar nutritional requirements. By contrast, co-occurring sub-communities show low MRO and little genetic relatedness: member species are neither particularly alike, nor do they particularly compete for the same resource. This discovery prompted the researchers to ask if it was possible that these species in fact produce and exchange complementary nutritional components and therefore gather in groups, much in the same way in which a team of professionals would work together towards a common goal and hire a new colleague whose skills complement theirs.
To express if, and to what extent, this is the case, the team devised another metric called metabolic interaction potential (MIP). The name is telling: by enumerating the metabolites each species provides, MIP describes a community’s ability to sustain itself through the exchange of metabolites. The higher a group’s potential for such exchange, the more the group as a whole benefits from what each member brings to it. To once again employ the workplace metaphor, MIP refers to what a team can achieve without outside help based on the individual skills of its members. The higher the number of different skills members bring to the workplace, the higher the benefit for the whole team. “It’s a win-win situation,” says Kiran Patil. “Bacteria obviously don’t make decisions as humans do but they still get selected into a community based on certain characteristics.”
Bacteria obviously don’t make decisions as humans do but they still get selected into a community based on certain characteristics.
It is one of the particular strengths of the new model compared to previous methods: it is able to make predictions about a community’s potential for exchange. Patil explains: “With microbial communities, currently people often just look at who is there, not how they interact. But rather than to only answer the question of “who is there?” our model can also answer the question of “what can they do, how can they interact?” What is so special about this research is that it shows that, although competition is unavoidable, the potential to cooperate can actually outweigh it as a driver of co-occurrence.”
At the same time, Patil explains, “the model answers questions about what is special about co-occurring groups. They can become very independent from the environmental supply of nutrients and therefore have a group advantage in nutrient-poor conditions which gives them better chances for survival.” The scientist compares the composition of such a community to a puzzle: it is precisely because each piece is different that they fit together to form a bigger picture.
“The main idea for the new method,” Patil recalls, “was born on a sunny day in a discussion between me, Aleksej, Sergej and Olga” – three of Patil’s co-authors – “over coffee on the rooftop of EMBL’s ATC building.” The building, fittingly, is used for training and conferences. “When we first had the idea,” Patil continues, “we thought it might be too challenging (to implement). But then we realised that, with some programming tricks and by taking advantage of large supercomputers, this can actually be achieved. We are now able to enumerate all possible pathways a nutrient can take in a considerably large community.”
We are now able to enumerate all possible pathways a nutrient can take in a considerably large community.
In the future, the method will be applied to three specific bacterial communities that are being studied at EMBL: the gut microbiome, data from the Tara expedition that from 2009 to 2012 gathered samples from the world’s oceans, and the fermented milk communities living in the drink Kefir. In doing so, the scientists are hoping, for example, to better understand the microbial diversity in the human gut. Applying the new model to these communities could shed some light on unanswered questions about how communities form and how the species in them cooperate and compete.
Looking for past print editions of EMBLetc.? Browse our archive, going back 20 years.
EMBLetc. archive