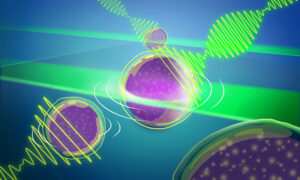
Actin crosslinking plays key role in tissue morphogenesis
In vivo study in Drosophila provides new insights into mechanisms behind embryonic development

Organismal development is characterised by changes in cell shape that are driven by physical forces acting on the cortical actin cytoskeleton of individual cells. These forces are tightly controlled in space and time, and are driven by the interaction between actin filaments and myosin motor proteins.
Using a combination of biochemistry, electron microscopy and optogenetics – the use of light to control the behaviour of cells in living tissue – scientists in the De Renzis and Sachse groups studied the mechanisms of action of a protein known as Bottleneck at the onset of embryogenesis in the fruit fly Drosophila. They discovered an important function of this protein in regulating the contractility of cells during tissue development. Although the Bottleneck protein itself is known only in Drosophila, the findings – published in The Journal of Cell Biology – point to a more general principle underlying the regulation of animal development.
A little-studied but important protein
Few studies have focused on the Bottleneck protein to date: it is expressed only for about 30 minutes at a particular stage of Drosophila embryogenesis, it is not encoded in the genomes of other animals, and its disordered structure and insolubility make it difficult to work with. So why bother?
Stefano De Renzis explains: “The early Drosophila embryo develops as a single large cell in which nuclei divide until they reach a critical density and individual cells start to form [a process known as cellularisation]. In the bottleneck mutant phenotype, which was identified over 20 years ago in the lab of EMBL alumnus and Nobel Laureate Eric Wieschaus, the cell constricts too early and nuclei become trapped inside constricted actin rings, resulting in very short cells [see video below]. The embryo does not develop, and eventually dies. Knowing that Bottleneck has a very important function during morphogenesis of the Drosophila embryo was sufficient justification for further study.”
Confocal video of two cellularising embryos viewed from the top (upper panels), and in cross-section (lower panels). Nuclei are show in cyan, and actomyosin fibres in white. In a Bottleneck/Filamin mutant embryo (right panel), the disorganisation and premature contraction of the actomyosin network caused an arrest of cellularisation apically of the nuclei. A wild-type embryo is show in the left panel. Scale bars: 20 µm. VIDEO: Daniel Krueger, De Renzis group/EMBL.
Actin crosslinking in vivo

When, with some perseverance, the scientists purified the Bottleneck protein, they showed that it functions as an actin crosslinker (figure 1), making actin fibres stiffer and more resistant to myosin forces, thus preventing premature contraction of the cells. Using optogenetic stimulation of myosin activity, they found that hexagonally arrayed actomyosin fibres (a complex of actin and myosin that comprises the contractile protein filaments of muscle tissue) seen during early cellularisation do not respond to myosin activation. Once the fibres acquire a ring conformation, they become contractile and respond to myosin. This transition is controlled by the induction of bottleneck gene expression.
The findings suggest that although Bottleneck is a Drosophila-specific protein, its mechanisms of action may be conserved and encoded in other proteins that belong to the class of crosslinkers. Thus, the gene and the protein may be different in other animals, but the process of actin crosslinking is the same. By studying a specific protein in a specific process, some general principles emerge regarding the regulation of tissue mechanics during embryogenesis. They point beyond a gene-centric framework for understanding morphogenesis, to more generally conserved biochemical processes.