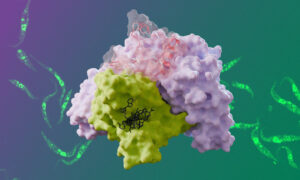
EMBL Hamburg lights the way to folding next-level origami
Recent findings artfully explore potential to transform proteins into custom-made structures
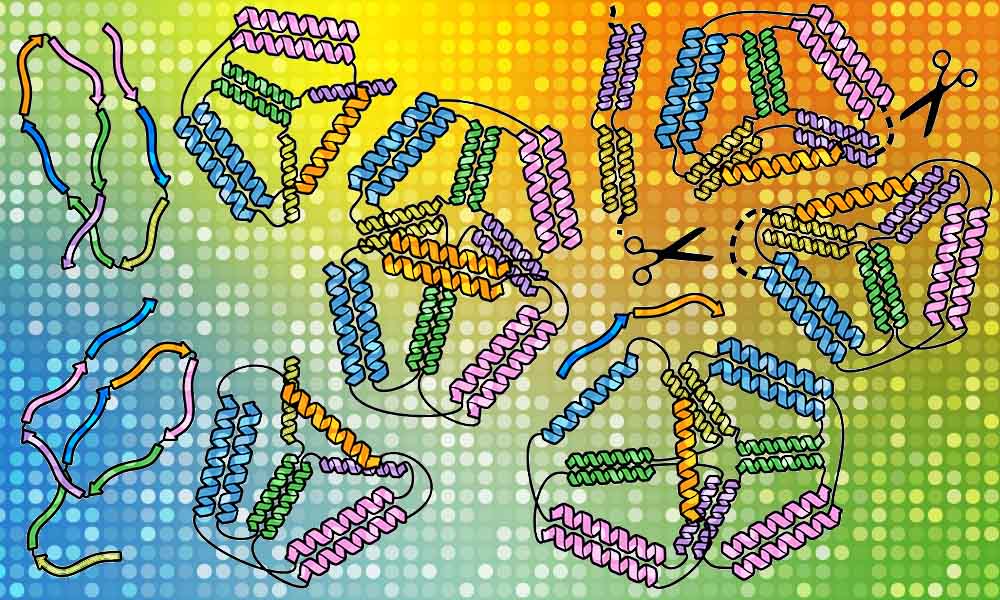
Origami may sound more like art than science, but a complex folding pathway that proteins use to determine their shape has been harnessed by molecular biologists, enabling them to build some of the most complex synthetic protein nanostructures to date.
Using EMBL Hamburg’s world-class beamline P12 at DESY’s PETRA III synchrotron, a team of Slovenian researchers, in collaboration with EMBL’s Svergun group, directed powerful X-ray beams at artificial proteins called coiled-coil origami. The proteins were designed to fold into a particular shape based on short modules that interact in pairs. By determining their molecular structure at the EMBL beamline, the researchers confirmed that the proteins folded into the desired shape and then studied the self-assembly process step by step. These findings advance understanding of how synthetic origami-like protein folding could potentially convey therapeutics, making it possible to more precisely target medication, minimising side-effects and maximising effectiveness.
“Recently scientists realised that natural proteins represent only a tiny fraction of possible protein shapes and that we can use design principles distinct from natural proteins. We can tailor designed proteins to make new materials, deliver drugs and vaccines, and much more,” says Roman Jerala, a synthetic biologist at the National Institute of Chemistry in Ljubljana, Slovenia, who led the work to design and build a bipyramid (a diamond shape made of two conjoined triangular pyramids) from different types of artificial protein chains.
While scientists first attempted origami using DNA, proteins lend themselves more to potential applications. Proteins are the molecular machines of life, containing long chains of amino acids that fold into shapes specific to the functions they serve. That can mean bolstering immunity, talking to other cells, or carrying out other tasks to keep the body healthy. The proteins used in this study were folded into braided ropes called coiled coils, which readily bind to other parts of the same chain or to other molecules. This makes them a particularly good building material for creating custom-made nanostructures.
Roman’s team first succeeded in this quest with a simpler origami structure – a single pyramid with a triangular base. They checked one chain of protein built of amino acids in a specific order and saw how it self-assembles. Then it was time to transform it from one structure to another, as if an origami lotus could transform into a crane. They put together two different chains of amino acids carrying a signal for a scissor enzyme called a protease, informing it where to make a cut in the origami protein. By doing so, they managed to force the protein to perform its origami transformation into a different shape.
Shining a light on protein solutions
To do this kind of work, the researchers need high-tech tools. EMBL Hamburg’s beamline P12 is particularly suited for this purpose, and EMBL’s Svergun group is world-renowned for its expertise in a technique called small-angle X-ray scattering (SAXS). Since 2018, EMBL scientists have been collaborating with the group from Slovenia, supporting them in using SAXS to study the structure of origami proteins.
“In SAXS, we shine X-rays at a glass capillary that contains the protein solutions. As the X-rays scatter as they pass through the solution, we have a way to interpret the structures,” says group leader Dmitri Svergun. “Here, most data collection work is automated, and we also provide important software and analysis support in collaborations like this one.”
Using the EMBL beamline, together with electron microscopy, calorimetry, computational modelling, and other methods, the researchers assembled the data needed to identify the structures of the origami proteins and confirm that the shapes would fit into their overall origami design.
“It’s our job to obtain the best signal from the beamline and create the optimal conditions for getting data,” says Stefano Da Vela, a postdoc in the Svergun group. “We provide tools to help make sense of the SAXS experimental data and create 3D models from the data.”
The researchers observed that their synthetic proteins assemble ‘bottom up’, which means that small, detailed bits form first and then assemble together into a bigger structure. Understanding this will help researchers construct more complex protein origami structures with more precision. “SAXS analysis was crucial in identifying which design leads to the desired shapes, and the superb tools developed at EMBL allowed us to detect unique features of our designed cages,” says Fabio Lapenta, a postdoc at the National Institute of Chemistry and the lead author of their recent paper in Nature Communications that described this work. “Coiled coils are excellent tools that can be used in cells as well as in isolated proteins. We think we can expand the potential of coiled-coil protein origami to design many new protein folds and introduce interesting functionalities.”