Taken out of context
In 1631, the printers tasked with reprinting the King James' Bible made a disastrous typographical error. They failed to spot a missing 'not' in one of the Ten Commandments, leading to a Bible that pronounced: "Thou shalt commit adultery." Hauled in front of a furious King and Archbishop, they were deprived of their printing license and fined. The offending copies of this bible, known today as the Wicked Bible, were hunted down and destroyed.
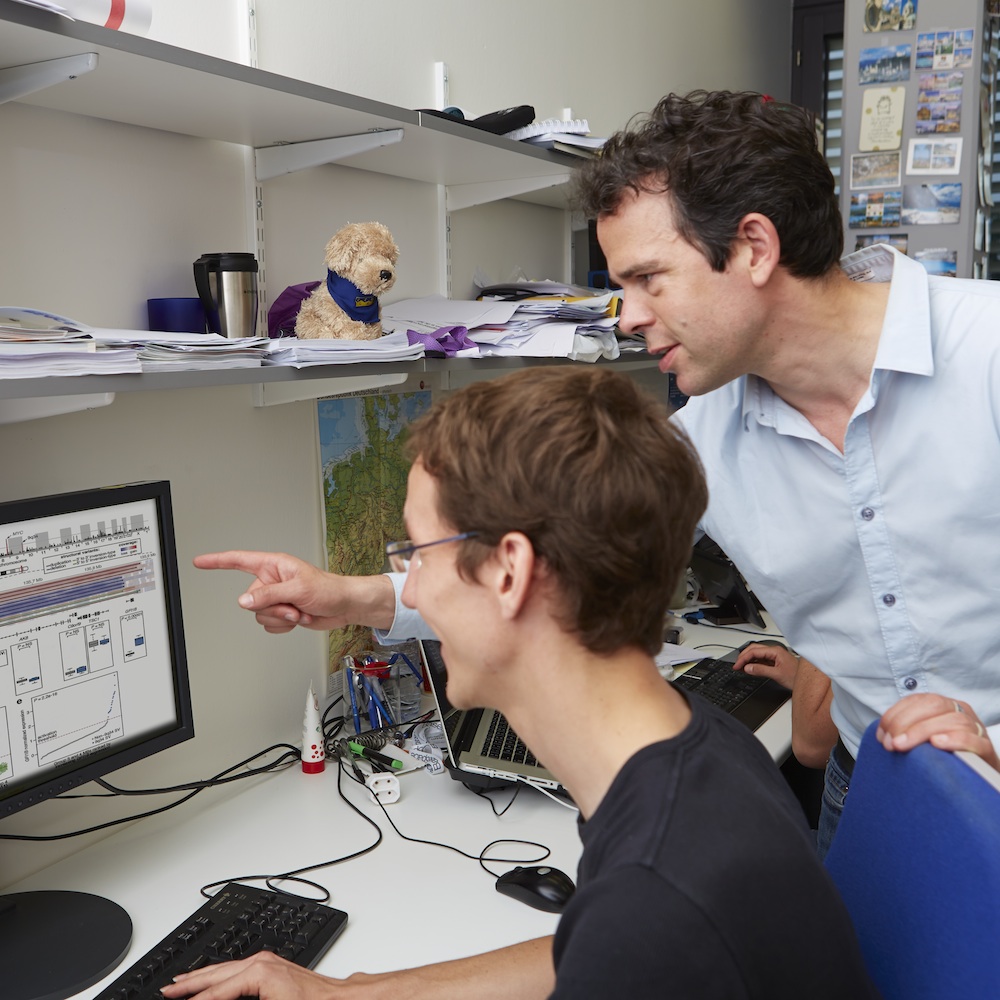
This story is a classic example of how a small change to the construction of a sentence, such as losing or changing a word, moving words to different places, or altering punctuation, can dramatically change our interpretation of the information contained within it. When it comes to meaning, context is everything.
The same is true of the genetic “words” in our DNA that are our genes. The way a cell uses the information contained in genes is heavily influenced by their context, such as their location on a chromosome. The biological equivalent of punctuation, the physical and chemical changes to chromosomes that tell the cell how genetic information should be read, is also key.
Like written sentences, our DNA can also suffer from typographical errors that disrupt chromosome structure and “punctuation”. The consequences can be severe. If genes are lost, altered, relocated or misread, this can lead to serious illnesses such as cancer. Now, Jan Korbel’s team at EMBL Heidelberg, in close collaboration with colleagues at the German Cancer Research Centre (DKFZ), have discovered how changes in a gene’s location and context help to drive the development of medulloblastoma, a deadly kind of brain cancer that affects children.
Genes can shuffle their position within the genetic code, or DNA, through changes to the structure of the chromosome to which they belong. These can happen by accident when chromosomes copy themselves, and can result in sections of DNA moving to new places (translocations), being turned upside-down (inversions), copied (duplications) or simply disappearing (deletions). This switching around can dramatically alter the instructions coded in that section of DNA. A duplication, for example, would look something like this something like this; inversion would look siht ekil gnihtemos. Other variants include translocations, where the wrong place pieces of DNA break off and get moved to in the chromosome.
These changes to the DNA are known as structural variants and have, until recently, been difficult to detect with the DNA sequencing methods used to analyse the human genome. While working as a postdoc at Yale University, Korbel developed a new technique called “paired-end mapping” that allows researchers to spot structural variants much more easily. When he joined EMBL five-and-a-half years ago, his group soon began applying, and further refining, this technique to study how structural variants cause genetic differences between individuals. But Korbel’s group soon also developed an interest in cancer cells and the changes to their chromosomes, which are notorious hotbeds of structural variation. Cancerous cells often end up with the wrong number of chromosomes, or chromosomes that appear grossly abnormal when inspected under the microscope. Korbel and his group wanted to find out whether smaller structural variants – that are much more abundant but which cannot be seen with a microscope – are also implicated in the disease, how they arise and what effect they have on cells.

They decided to focus on medulloblastoma, a rare but deadly form of brain tumour that affects children. “There are no suitable treatments to combat the disease, at least for the majority of patients,” says Korbel. One of the key barriers to the development of treatments is that medulloblastoma tumour cells seem to arise very differently to those from many other cancers. Tumour cells often contain combinations of changes, or mutations, to their DNA sequence that are characteristic of a specific cancer, and can therefore be used to aid diagnosis and treatment. These mutations can make a gene – or the protein it encodes – hyperactive, causing the cell to behave abnormally. Many modern anti-cancer drugs target these pathways, such as the leukaemia drug Gleevec.
But few such mutations have been found in medulloblastomas. Based on their biology and clinical symptoms, doctors have grouped the tumours into four different types, and for two of these – group 1 (also known as the WNT-group) and 2 (also known as the SHH-group), some mutations have been identified. But the underlying genetic causes of groups 3 and 4 were mysterious.
Researchers already knew that structural variations are present in group 3 and 4 medulloblastomas, but had little idea of what these variations might be doing. This, together with the fact that Korbel’s group had a long-standing collaboration with Stefan Pfister at the German Cancer Research Centre (DKFZ) in Heidelberg, led them to investigate further. “It made a very nice case for a strong collaboration, as we could join our group’s expertise on understanding variation in the genome with Stefan Pfister’s strong clinical research expertise,” says Korbel.
The team sequenced the genomes of medulloblastomas from patients and found a region on chromosome 9 that harboured structural variations. The variations took different forms in different patients: some had inversions, some had deletions, others had duplications, and still others had more complex changes. But they all had one thing in common: the variations all resulted in a gene called GFI1B becoming abnormally active.
But unlike overactive genes in other cancers, the DNA of the GFI1B gene in the medulloblastoma cells was normal. Its sequence had not altered; all that had changed was the gene’s location, similar to a word being in the wrong position in a sentence. Further investigation revealed that GFI1B’s new location contained chemical and physical “punctuation” that encourages the cell to use the information contained in the nearby genes.
The process we uncovered here may be much more prevalent in solid tumours.
This punctuation relates to the way genomic information is packaged into chromosomes. DNA is wound around proteins called histones, which both offer protection and help control gene activity. Genes that are supposed to be inactive are wound tightly around histones to stop the cell’s gene-reading machinery from accessing the DNA sequence of that gene. By contrast, the DNA that contains active genes is much more loosely wound, allowing the cell to read the information contained within. Because these changes affect gene behaviour rather than its sequence, they are known as ‘epigenetic’ effects.
In brain cells, the GFI1B gene is usually switched off, its DNA packed away tightly. But in the medulloblastoma cells, Thomas Zichner, a postdoc in Korbel’s group, found that regions of DNA containing GFI1B had been moved to another part of the chromosome, next to regions known as ‘enhancers’ that can promote gene activity. Enhancers encourage the DNA and histones with which they come into contact to become more relaxed and open and the genes within them more active. “For us, they are the most plausible culprits for the overactivation of the gene,” says Korbel. To prove this beyond doubt, the team will need to conduct further experiments, he adds.
Although similar processes have been seen in various leukaemias, this is the first time such a mechanism has been described in solid cancers. “The process we uncovered here may be much more prevalent in tumours,” says Korbel. This could give researchers new insights into how hyperactive genes drive cancer. It could also give them a new approach to study tumours in which the genetic drivers are unknown, and highlights how the interaction between a gene and its epigenetic environment can make all the difference it its behaviour.
Listen to Jan discuss why cancer researchers don’t usually look for this kind of gene activation: