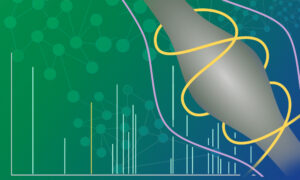
Read the latest Issue
What CRISPR may bring for the future of biology, and how it is used at EMBL
One of the most popular metaphors used to describe CRISPR-Cas9 – often referred to simply as ‘CRISPR’ – is that of a pair of genetic scissors: a cut-and-paste tool at the molecular level. Based on an ancient bacterial defence mechanism, its enormous potential for gene editing has only been discovered during the last decade and has revolutionised biology. CRISPR is built from an individually designed RNA strand and a DNA-cutting protein, Cas9 (see infographic, p. 19). Three important qualities make this tool invaluable for the life sciences: it is cheap, easy to use and very precise. With CRISPR it’s possible to target any known DNA region and deactivate a gene or introduce a new one. From medical therapy to fundamental research, CRISPR has a large range of applications and is used by research groups at EMBL to address important questions in biology.
To what extent do mutations in cancer cells interact with one another? That’s one of the questions that EMBL’s Korbel group is trying to answer using CRISPR. “This is the principle of epistasis. In other words, we want to know whether one mutation in cancer can affect another mutation and if there is synergy between them,” says group leader Jan Korbel. This is done by using cell lines originating from the same donor, but with different gene mutations. By deactivating a gene using CRISPR, the scientists can observe how this affects other genes.
“We also reconstruct fairly large-scale rearrangements in the genome that we have previously predicted to have a functional effect,” says Korbel. “Here we use the gene scissors to break the genome and remake it. The attempt is to repeat what we have observed in a disease. We make these rearrangements and anticipate a functional effect on gene expression.”
CRISPR is a very versatile tool and can be used in all types of organisms, from yeast to mammalian cells. EMBL’s Steinmetz group uses this flexibility to their advantage. “We do a fairly broad coverage of the CRISPR-Cas 9 landscape,” says group leader Lars Steinmetz. “We use it to study natural variants, to do genetic screens, and we think about how it could be used more safely for therapeutic editing applications.”
The Steinmetz group studies the impact of single-nucleotide polymorphisms (SNPs) on phenotypes – that is, an organism’s observable characteristics. SNPs are cases where a single DNA base has been substituted for another – a T instead of a C, for example – at a specific location in the genome. SNPs can have biomedical effects, such as increasing susceptibility to certain diseases or affecting the body’s response to treatment. To study the effects of SNPs on an organism, the Steinmetz group carries out large-scale experiments. “CRISPR enables us to do that with high efficiency. We developed an approach where we can engineer 10,000 to 100,000 different SNP variants in genomes of yeast,” says Steinmetz. One goal of the project is to improve the methodology of CRISPR editing by increasing the efficiency of DNA repair at the cut site. This makes it more probable to get the desired edit.
Another project in the Steinmetz group involves research on off-target edits – unwanted side-effects of CRISPR gene editing. At the stage when a mouse embryo consists of only two cells, they modify one of these cells using one of several different CRISPR editing techniques that are under investigation. The genomes of the cell populations derived from these two cells are then compared. This makes it possible to determine which mutations are natural background mutations and which are caused by off-target edits. The number of off-target effects reveals the accuracy of different editing methods and makes it possible for the researchers to start finding ways to improve them.
The fruit fly – Drosophila melanogaster – remains a model organism of choice for biological research, and is widely used at EMBL. Alessandra Reversi, a research technician in the Ephrussi group, provides gene-editing services in fruit flies, creating genetically modified strains for scientists across EMBL.
“Using CRISPR is the best strategy for genome editing,” says Reversi. “The advantage of CRISPR is that you can now modify the Drosophila genome with even single-nucleotide precision. Compared with previous strategies, editing the fruit fly genome with CRISPR is more precise, and much easier, faster and cheaper. With this technique, scientists no longer need to perform time-consuming screens in order to identify flies with the desired modifications.”
Fruit flies are not the only organisms for which EMBL provides gene-editing services. EMBL Rome has a Gene Editing and Embryology Facility that uses CRISPR to provide the whole of EMBL with gene-editing services in mice.
A relatively unusual application of CRISPR is epigenetic editing, which is being carried out in EMBL Rome’s Hackett group by postdoc Cristina Policarpi and PhD student Valentina Carlini. Epigenetics refers to the chemical changes that affect gene expression without actually altering the DNA sequence. One key type of epigenetic change is DNA methylation – the addition to the DNA molecule of a chemical group containing one carbon atom and three hydrogen atoms. Other types of epigenetic change involve chemical modifications of histones – the proteins around which our DNA is wrapped.
The Cas9 proteins used in Policarpi and Carlini’s research have been modified so that they are unable to cut DNA: instead, they recruit other proteins. These recruited proteins then alter the epigenome through DNA methylation or histone modifications. “The main goal of the project is to understand how all these epigenetic marks contribute towards gene expression and genome organisation,” says Policarpi. “We add or remove epigenetic marks only in small parts of the genome and we don’t touch anything else, so we’re sure that we’re studying only the contribution of those marks to gene expression and regulation.”
In another project in the Hackett group, CRISPR is used to knock out single genes in a pool of mouse cells – a different gene in each cell, until the 20,000 genes in the mouse genome have been covered. This makes it possible to study the effects of the absence of any gene on a phenotype of interest. An experiment on such a large scale is only possible with a tool like CRISPR. Policarpi has to laugh: “Otherwise you would spend your whole life and that of your children and grandchildren in the lab!”
There are still problems to overcome in the use of CRISPR. “The main concerns are editing at high efficiency and the issue of on-target editing accuracy,” says Steinmetz. “You have to worry about off-target effects: apart from what I wanted to achieve, what else did I do to the cell by manipulating the system and introducing a new enzyme? These concerns get really important when you want to go into any form of therapeutic application.”
Political and public concerns focus mostly on germline gene editing with CRISPR, where genes are edited so early in an organism’s development that the changes are copied into all future cells, including sperm and egg cells. This means the edited genes will be passed on to future generations. These concerns have spiked since the case in November 2018 in which Chinese researcher He Jiankui claimed to have edited the genomes of two human embryos, using CRISPR to introduce a gene that would increase HIV resistance. This action was widely condemned by the scientific community as an experiment on humans that could have potential off-target effects, while its benefits remain questionable.
“There’s of course a huge ethical debate about whether one should do germline editing in humans,” explains Korbel. “My standpoint and EMBL’s standpoint is that this should not be done. Introducing changes into a human being before he or she is born creates a huge issue of consent, as these mutations would remain in the germline for future generations, with consequences that are not clear. My position would be to have a moratorium for some years to come, to better understand the rates of errors that are introduced during gene editing with CRISPR, and to have a full ethical discussion.”
Ewan Birney, director of EMBL’s European Bioinformatics Institute (EMBL-EBI), agrees that this type of science should be closely regulated. He points out that, in many countries, couples who carry a serious genetic disease and who are conceiving by in vitro fertilisation (IVF) can choose to implant only those embryos that do not carry the disease. This procedure is called pre-implantation genetic diagnosis, and is subject to strong national regulation. “The CRISPR approach would add an extra step of introducing CRISPR technology at the first-cell stage,” Birney explains. “This would be followed by screening for a successful edit. Although this is technically possible, there are currently virtually no serious genetic diseases where pre-implantation diagnosis would not work but CRISPR would.”
Birney highlights that society must be able to trust in legislation to guarantee the ethical use of a new technology like CRISPR. “In the UK, the Human Fertilisation and Embryology Authority provides regulation enshrined in UK law in this area. Other countries in Europe have analogous legislation and regulation. This allows new technologies, such as mitochondrial donation [see pp. 50–51], to be developed in a way that is medically safe, scientifically and ethically sound, and supported by society.”
One problem of national regulation is that technology is advancing at a rapid pace and political decisions are not keeping up. “The legal framework in Germany is fairly old,” says Korbel. “The Embryonenschutzgesetz, the embryo protection law, was written prior to the discovery of CRISPR, so the set of techniques and tools that scientists now have in their hands is way beyond what the legislators anticipated. That implies that the law should be reviewed.”
While the prospect of eugenics or designer babies in the near future is unsettling, CRISPR itself is only a tool and therefore its effects depend on how responsibly – or otherwise – it is used. “There is still the potential to fix thousands of mutations that affect somatic cells in the human body. These diseases could be eliminated or prevented before they occur,” says Steinmetz. “It’s fascinating how this technology can work across systems, in a mammalian cell as well as in yeast or bacteria. The potential is enormous and I have no doubt that it’s going to change the way we think about our healthcare, the kind of food we eat, the way we live.”
If you are a journalist and wish to arrange an interview with any of the scientists featured in this article, please contact pressoffice@embl.de.
Looking for past print editions of EMBLetc.? Browse our archive, going back 20 years.
EMBLetc. archive